X-Ray Spectrometer (XRS)
Schematic diagram of the XRS system is shown below (postscript version is also avilable).
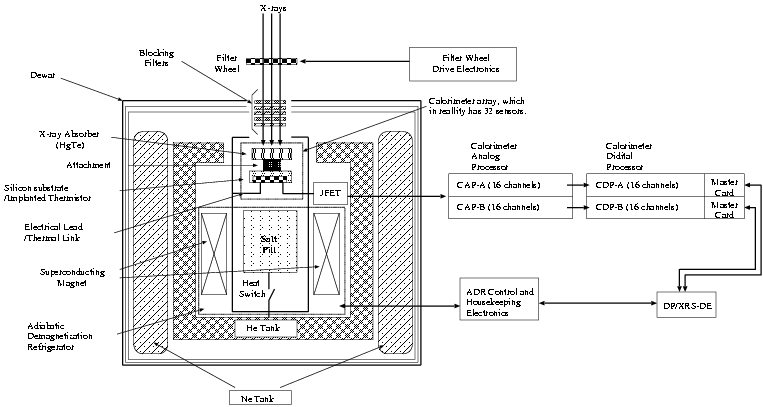
XRS Instrument
Calorimeter Array
X-ray calorimeters measure the energy of the incident X-ray photon by determining temperature of the absorbing material, which has to be sufficiently opaque to X-rays. Energy resolution of the calorimeter is higher for lower heat capacity of the absorber. In Suzaku XRS, mercury telluride (HgTe) was chosen as the absorber to satisfy these requirements.
The calorimeter array has 30 active pixels in a 6x6 geometry. The 4 corner pixels are unused, and two further pixels are inactive. Instead, there is a pixel dedicated to monitoring the on-board calibration source. Each pixel size is 625 x 625 microns, covering roughly 2.9'x2.9' area on the sky (see the schematic).
On each pixel, the HgTe absorber is attached on the monolithic silicon substrate in which the thermistor is ion-implanted. The thermistor changes its resistance with the temperature in the range of 10-50 mega-ohms, and as a result the temperature increase is detected as a change of the voltage across it. Signal from each thermistor is picked up by the JFET and sent to the Calorimeter Analogue Processor (CAP) individually. CAP provides power to the detector and amplifies the signals by a factor of 20,000. CAP has 32 channels, each of which handles data from a single calorimeter pixel.
The detector assembly needs to be kept at 60 mK within accuracy of tens of micro-Kelvins to operate at the maximum performance. The rise time of the temperature is a few millisecond, and the total recovery time is about 80 ms, which determines the maximum counting rate of the observable sources.
Beneath the calorimeter array, a silicon PIN anti-coincidence detector is put in order to detect background particles which penetrate the calorimeter array. XRS events which are simultaneously detected with the anti-coincidence detector are flagged and may be rejected in later data analysis.
Cryogenics and Refrigerator
The instrument is contained in the insulated dewar, which consists of nested shells. At the center of the dewar is the helium tank filled with 25 litter liquid helium whose temperature is about 1.5 K. It is surrounded by the neon tank which is filled with 100 litter solid neon at a temperature of about 17 K. The time required for all the solid neon to completely melt determines the life-time of XRS, which is expected to be 2-3 years.
The XRS detector assembly (named Front End Assembly - FEA) is placed inside the helium tank and is thermally connected to the Adiabatic Demagnetization Refrigerator (ADR), which cools the FEA down to 65 mK. The ADR works first by aligning the magnetic spins of the molecules in the salt pill using a superconducting magnet. The salt pill is then connected to the liquid helium bath via the heat switch, allowing it to cool down to the liquid helium temperature. Once it has reached equilibrium, the heat switch is opened, and the magnetic filed is reduced to nearly zero. The spins of the salt molecules are thereby allowed to flip in random directions to reach a high entropy state (adiabatically demagnetized). The heat to increase the entropy is absorbed from the salt pill, thus the salt pill, and the detector, is cooled. The Suzaku ADR can keep the detector at the desirable temperature for about one day. Eventually, all the magnetic spins get completely random, and no more heat can be absorbed. Then the magnetic field is increased again. The "recharge" of the refrigerator, typically lasting about 60 minutes, will be performed once every ~20 hours. The recharge process will preferentially be carried out when observing targets are occulted by Earth to minimize the loss of observing time. The temperature is maintained at the desired level within tens of micro-Kelvins, by carefully adjusting the magnetic field change cycles via a feedback loop. ADR is controlled by the ADR Control and Housekeeping Electronics (ACHE). ACHE cycles the ADR and control the ADR temperature at the desirable level.
Blocking Filters
There are five blocking filters made of extremely thin sheets of aluminized polyimide, which shields the detector from the space. They are put at the entrance window of each shell of the dewar, and named from outside to inside, DMS (Dewar Main Shell) filter, IVCS (Inner Vapor Cooled Shell) filter, Neon Filter, FEA (Front End Assembly) filter, and CTS (Calorimeter Thermal Sink) filter. CTS is the part attached to the cold end of the ADR, and the CTS filter is directly on the lid of the detector box. The blocking filters let the X-rays through to the detector, but keep out other radiations which can be a source of the heat and noise. The transmission of the filters determines the lower energy boundary of the XRS energy range. It is expected XRS will have a high enough throughput down to ~0.3 keV.
Calibration Pixel
The XRS includes an on-board calibration pixel to track variations in the gain, which could be caused by a drift in the temperature. The calibration pixel is offset several millimeters from the imaging array, and illuminated by a collimated 55Fe source (the 30 pixels that observe the sky do not see this source). The decay of 55Fe to 55Mn produces 5.899 and 5.88 keV (Kalpha1 and Kalpha2) and 6.490 keV (Kbeta) lines with a half-life of 2.73 years. The count rate of the calibration pixel will be ~4.5 counts/s at launch.
Filter Wheel
XRS has a rather large deadtime of ~80 msec. Consequently, in order to observe bright sources with XRS, the X-ray flux is desirable to be reduced to avoid event pile-up. The Filter Wheel is put in front of the XRS to this end. The Filter Wheel is a circular plate made of molybdenum with six windows.
There are three types of filters: open, 300 micron beryllium, and neutral density. The neutral density filters are 200 micron thick molybdenum plates that have been mechanically drilled to allow 10% of X-rays to pass through. For each type of filters, one window also contain 55Fe (and in the case of one of the open filters, also 41Ca) isotopes to allow direct monitoring of gain stability in the imaging array.
Depending on the scientific requirements, appropriate windows are chosen and put in front of the XRS. The Filter Wheel Drive Electronics (FDE) rotates the Filter Wheel using stepping motors.
On Board Data Processing
Outline
When an X-ray photon is detected, the microcalorimeter generates a pulse signal, which is amplified by the JFET. Furthermore, CAP amplifies the signals by a factor of 20,000 so they can be transmitted to CDP (Calorimeter Digital Processor). Both CAP and CDP have two independent sides named Side-A and B. Each side has 16 channels, and the 32 channels and detector pixels have one-to-one correspondence. There are two Master Cards in CDP for Side-A and Side-B. CDP communicates with the spacecraft Data Processor (DP) through the Master Cards.
The job of the CDP is to extract X-ray events from large amount of the raw signals, determine the event arrival times and energies, and send compressed event packets to the spacecraft Data Processor (DP). Each channel of CDP consists of an antialiasing filter (or low-pass filter), A/D converter and Digital Signal Processor (DSP). The antialias filter cuts off the frequency above 2 kHz. DSP samples and digitizes the data at a rate of 12288 Hz with 14-bit resolution. For High Resolution mode (see the next section), 2048 samples are required to analyze a single pixel. Therefore, the amount of raw data in a single pulse is 2048 samples x 14 bits/sample= 28672 bits. DSP determines the pulseheight and arrival time, and outputs the event packet along with other information. A single event packet to output is 64 bits in length, which favorably compares to the amount of the raw data.
Telemetry limit for XRS is 10240 bits/sec for the total 32 pixels, which corresponds to 160 events/s/32 pixels, as a single event XRS consumes 64 bits. Also there is a hardware limit of the XRS counting rate, which is 50 counts/s/pixel.
Pulse Detection
The first job of the DSP/CDP is to detect X-ray pulses in the data stream. To do this, DSP calculates smoothed derivative of the data. The initial pulses are detected when the derivative exceeds a fixed threshold.It will be easy to detect initial pulses, but the harder part is to find secondary pulses which might be superposed on the tails of the initial pulses. If the secondary pulses are missed, this may affect the determination of the pulse heights of the initial pulses. Hence it is important for DSP/CDP to detect secondary pulses even if they are much smaller than the initial pulses.
To search for the secondary pulses, DSP/CDP compares the smoothed derivative with a template of the single-pulse derivative shape. The CDP software maintains a copy of what the single-pulse derivative shape is. The derivative shape template is subtracted from the measured derivative by scaling the peak values to form the adjusted derivative. If the adjusted derivative rises above a threshold and then falls below within a specified length of time (corresponding to the recovery time, ~80 msec), the secondary pulses are detected. The secondary pulses are flagged so that they can be discriminated from initial pulses.
Once an initial pulse has been detected, CDP begins counting down the length of a Hi-resolution data record. If the pulse-height reaches zero without detecting any secondary pulses within 2048 samples (=2048/12288=167 msec), the event is flagged as a Hi-res record. If a secondary pulse does occur, the initial pulse will be proceeded as either a Mid-res or Low-res event, and the counter will reset to the full Hi-res length.
Pulse Height Determination
It is one of the most important tasks for CDP to get the best possible estimate of the height of each pulse. The zeroth-order pulse-height is the peak value of the pulse minus the baseline value, but this is not a good measure due to the noise. In order to determine the pulse height value as precisely as possible using all the samples in the pulse, >i>optimal filtering technique is adopted. In this technique (named Hi-res mode), noise is reduced by averaging all the samples in the pulse. The Hi-res mode is usable only when there is a single pulse in the block of 2048 samples.To create the optimal filter, time series of the average pulse (2048 samples in 167 msec) is Fourier transformed, and in the Fourier space it is divided by the power spectrum of the noise, then inverse Fourier transformed. In order to estimate accurate noise power spectrum, a relatively large number (100-200) of individual noise power spectra, each of which is made from a single noise record of 2048 samples, are running-averaged. The average pulse is made by running-averaging similar pulses which fall in a limited range on the pulse-height vs rise-time plane. The optimal filtering template in the Hi-res mode thus has 2048 samples in time space. The optimal pulse height is calculated by multiplying the data and optimal filter by sample-by-sample and adding them up. This procedure cancels out the interference of the noise component in the pulse.
The above method of the pulse-height calculation in the Hi-res mode cannot be used when there are two or more events in the chunk of 2048 samples. If two pulses are closer than 2048 samples but not "too close," a shorter optimal filtering template, typically 512 samples long, is used. This method of the pulse hight calculation is called Mid-res mode. In general shorter templates are less effective to determine and reject the interference of the noise at particular frequencies. If there are no noise tones, the Mid-res pulses will approximately give the same resolution as the Hi-res mode.
When pulses are too close even for the Mid-res mode, pulse heights are determined simply measuring the heights of the peak over the baseline level. The baseline is measured by taking average of typically eight samples right before the start of the pulse.
Each XRS event has a flag which tells either of the three methods of the pulse height determination is used. Hi-res event mode provides a resolution that is limited by the detector and amplifier electronics, and this has been as good as 5 eV (FWHM) at 1 keV in laboratory. Mid-res mode provides better than 6 eV resolution at 1 keV. The Low-res mode gives a resolution around 30 eV.
For more information, please see the XRS page maintained by the GSFC XRS team.
If you have any questions concerning Suzaku, visit the Feedback form.